Controlled release systems
Development of controlled release devices aims at the regulated, prolonged delivery of drugs, agrochemicals or other bioactive agents.
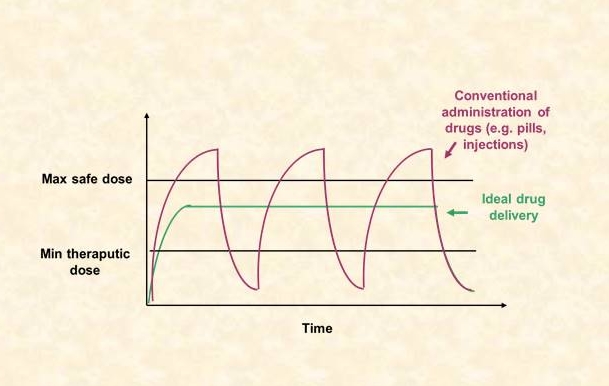
Matrix-type controlled release (MCR) devices consist of a polymer matrix incorporating the bioactive solute and are activated by the ingress of water when placed in aqueous biological environment. In comparison with other types of controlled release systems (e.g. reservoir systems or osmotic pumps), MCR devices are very attractive commercially, due to their structural simplicity and low cost of manufacture. Examples of marketed MCR medical devices include subdermal and ocular implants, transdermal “patches” and drug-eluting stents.1
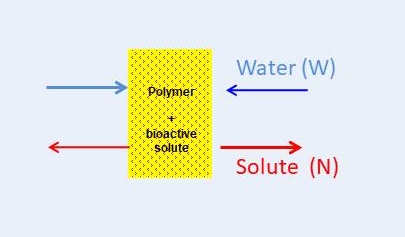
In the common practical case of fast solvent penetration, solute release kinetics follow a t1/2 law for a major part of the process time scale (as for example in the case of steroid release from single layer PDMS matrices). This leads to an undesirable large continuous decline of dose rate, which is particularly marked in the initial stages (“burst effect”). Elimination of the burst effect and achievement of uniform release rate becomes increasingly important especially for drugs with narrow “therapeutic range”. A major part of our work on MCR systems deals with the optimization of the design of such devices in this respect.
- Modeling of matrix-type controlled release (MCR) devices and experimental validation
- Modelling of single layer devices
The basis for modelling release kinetics are Fick’s equation for solute loads below the solute’s solubility limit in the matrix, and Higuchi’s equation for supersaturated matrices, where part of the solute is in the state of fine dispersion. Higuchi equation is strictly applicable to (i) a homogeneous fine dispersion of the drug particles in the polymeric medium (ii) fast solvent penetration in the matrix from the surrounding medium (usually aqueous) (iii) fast dissolution of the drug particles as compared to diffusion of the dissolved drug and (iv) the presence of the drug does not materially affect the degree of swelling of the matrix.
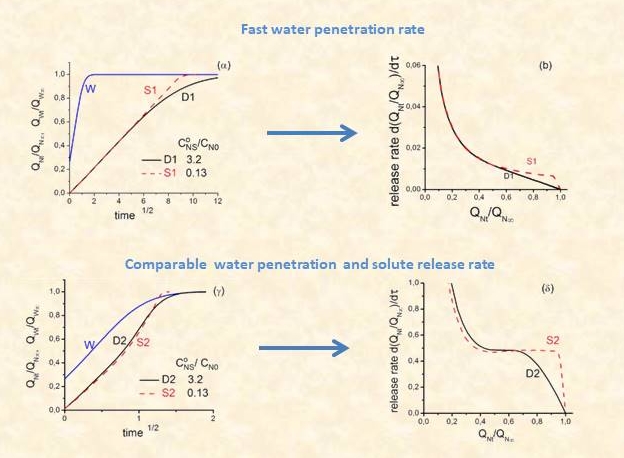
We developed a generalised model2,3 for MCR systems functioning under a much broader range of experimental conditions, than those described by the Higuchi model. It takes account for the effect of each diffusing species (solvent-solute) on the diffusivity and/or solubility of the other and can simulate the release performance of devices functioning under various experimental conditions. These include unsaturated or supersaturated systems, fast or comparable rate of water ingress in comparison to drug release, osmotic effects of embedded solute and slow macromolecular relaxation of glassy matrices in response to swelling stresses induced by the solvent.4
The model can serve as a tool for the (i) elucidation of the effect of individual MCR design factors on the rate of release and help develop design strategies for achieving a constant release rate (ii) predictive evaluation of the performance of proposed specific MCR devices.
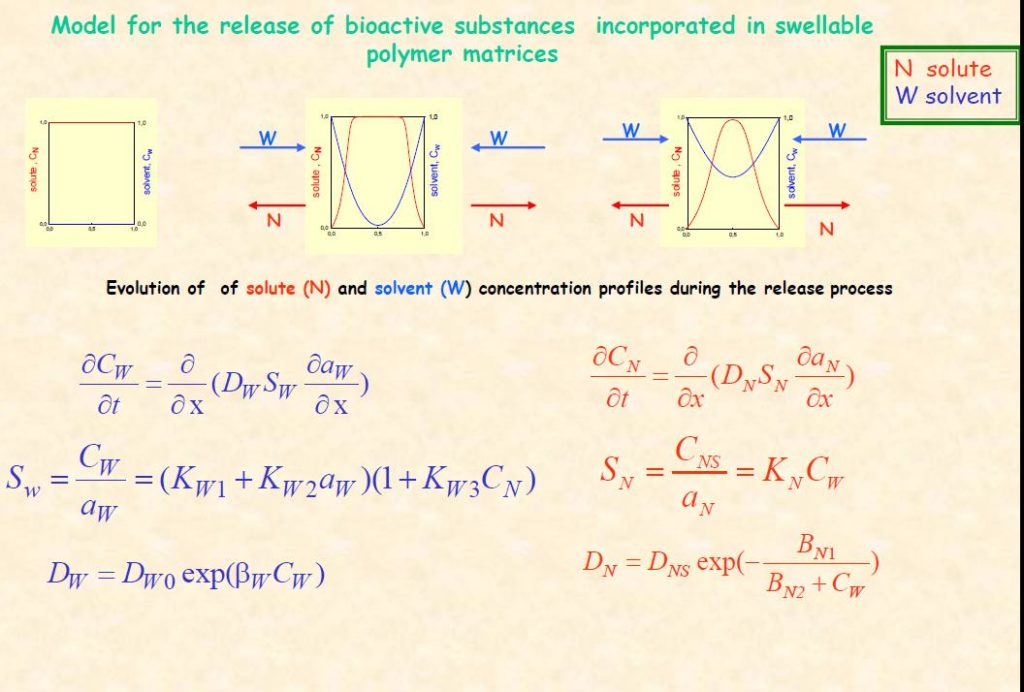
Validation of various aspect of the model against experimental release kinetics is performed through independent measurements of the transport properties (solubility-diffusivity) of the polymer –water and polymer-drug systems. These include model systems (cellulose acetate- NaCl,5,6 cellulose acetate-NaI) as well as pharmaceutical ones (polyvinyl alcohol loaded with propranolol7 or diphylline, PDMS loaded with xanthine derivatives8)
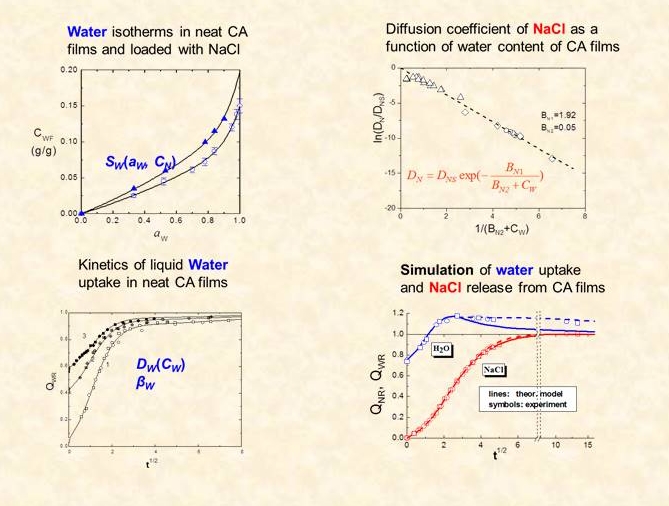
- Modelling of multilayer devices
The model was extended to three-layer symmetrical ABA matrices,9,10 which are used in practice mainly to eliminate the burst effect as for example in coated tablets.
Systematic parametric studies were performed in order to derive criteria for improved performance of MCR devices (i.e. improved constancy of the release rate and reduced burst effect)
Computations included ABA systems with layers A and B with
- Different initial solute loading
- Different permeation properties
- Different thicknesses , and
- Combinations of the above
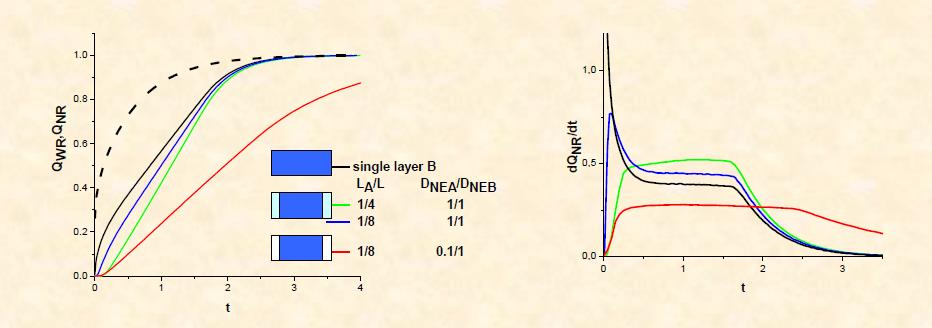
Using the same methodology as in the single-layer MCR cases above, the model was validated against experiment for theophylline release from PDMS and from composite PDMS-PEG matrices.8
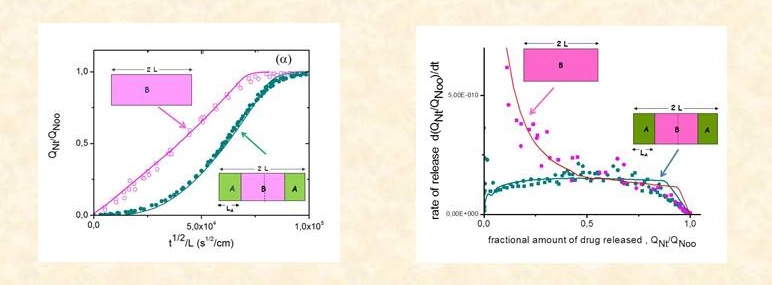
- Release mechanisms
In real MCR systems several factors, not included in the mathematical modelling approaches described above, affect release kinetics due to the finite size of embedded solute particles, to structural changes of the solute depleted matrix during the release process and to osmotic effects induced by hydrophilic solutes. To gain insight on the mechanisms of release we studied (i) a series of solutes ranging from sparingly to highly soluble inorganic salts embedded at different loads in cellulose acetate matrices (CA)11-13 and (ii) inorganic salts and a drug embedded in elastomeric PDMS matrices.14,15 Additional information on the systems studied were provided by (i) monitoring the water content of the matrix during the release process, (ii) comparison of the diffusion properties of particle-depleted matrix with those of the neat polymer, which is related to any rate-controlling irreversible structural changes in the depleted zone (iii) studying the mechanical properties of the loaded and the solute-depleted matrices. This work provided insight on several effects influencing the release kinetics, namely (i) the effect of incomplete encapsulation of solute particles in the matrix, illustrated be the case of sparingly soluble CaSO4 embedded in CA matrices11 (ii) the effect of mechanical rupture of the thin polymer wall encapsulating the solute cavity, as in the case of highly osmotic solutes in hydrophobic PDMS matrices14 (iii) a new mechanism of osmotically induced release, proposed by us, applicable to highly and moderately soluble solutes in CA matrices.12
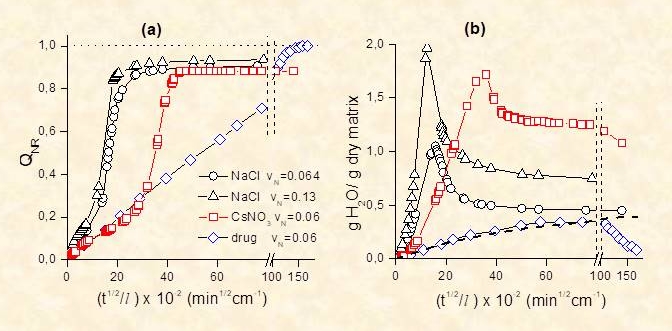
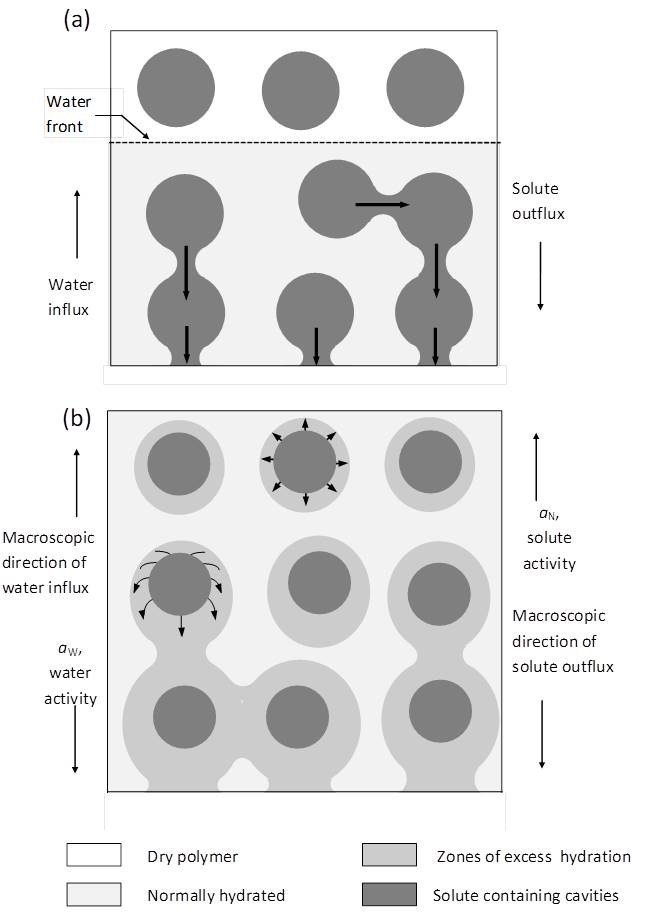
- Modification of polymer matrices
Modification of existing polymers used in CR systems is sought either (i) to facilitate the release of high MW and /or polar solutes (e.g proteins, growth factors, hydrophilic drugs) from hydrophobic matrices or (ii) to achieve stabilization of the release rate. Our work includes modification of hydrogels or elastomers by varying the swelling capacity of the matrix, through chemical (e.g. crosslinking of PVA hydrogels16 or grafting of hydrophilic moieties on PDMS elastomer17,18) or physical (heat treatment of PVA,16 or blending of PDMS with hydrophilic additives19-22) methods.
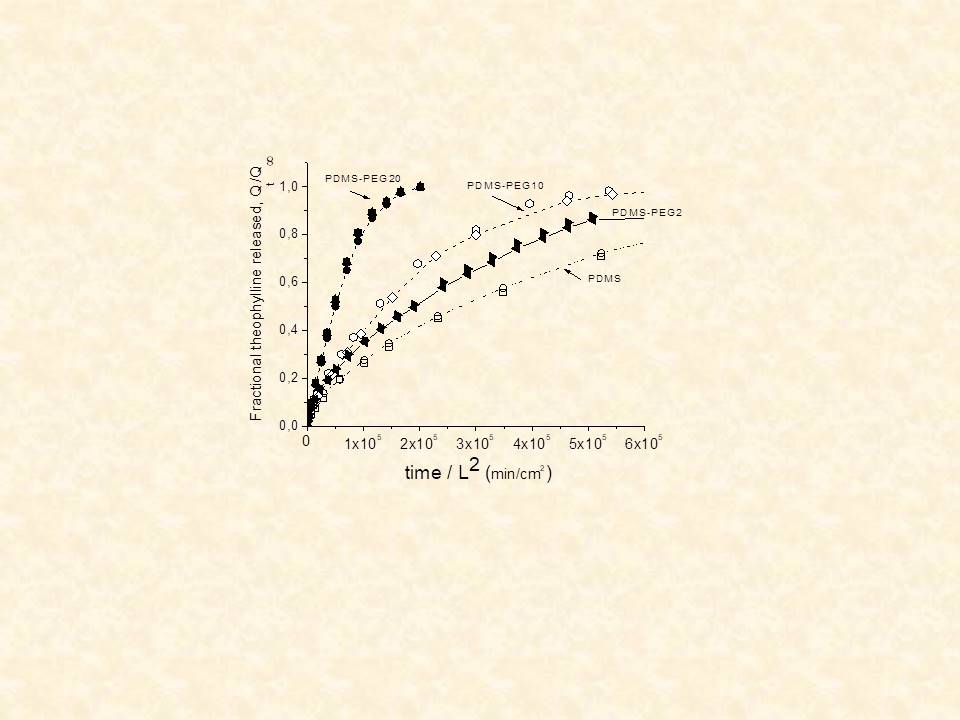
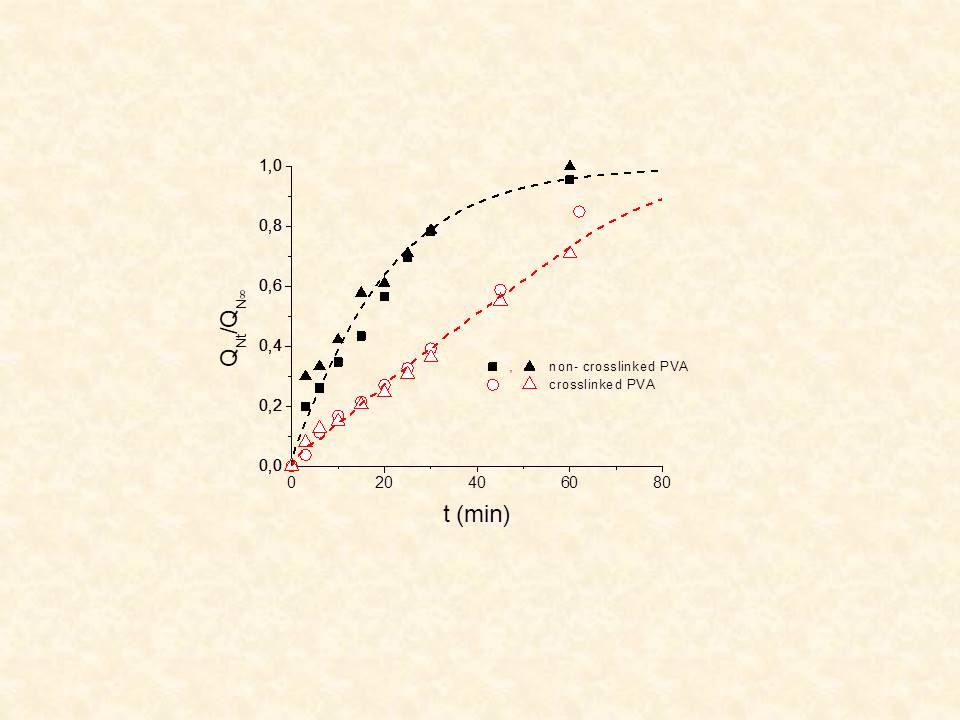
References
- M. Sanopoulou, K.G. Papadokostaki, Controlled drug release systems: Mechanisms and kinetics in Biomedical Membranes And (Bio)artificial Organs, Stamatialis D. (Ed.), World Scientific Publishing Co. Pte. Ltd., Chap. 1, 1-33 (2017)
- J.H. Petropoulos, K.G. Papadokostaki and S.G. Amarantos, A General Model for the Release of Active Agents Incorporated in Swellable Polymeric Matrices, J. Polymer Sci., Part B: Polymer Phys., 30 (1992) 717-725.
- J.H. Petropoulos, K.G. Papadokostaki, M. Sanopoulou, Higuchi’s equation and beyond: Overview of the formulation and application of a generalized model of drug release from polymeric matrices, Int. J. Pharm. 437 (2012) 178-191, mini review
- K.G. Papadokostaki, A.Ya. Polishchuk and J.K. Petrou, Modeling of Solute Release from Polymeric Monoliths Subject to Linear Structural Relaxation J. Polymer Sci. Polym. Phys., 40 (2002) 1171-1188
- K.G. Papadokostaki and J. K. Petrou, Combined Experimental and Computer Simulation Study of the Kinetics of Solute Release from a Relaxing Swellable Polymer Matrix. Ι.Characterization of non-Fickian Solvent Uptake J. Applied Polym. Sci. 92 (2004) 2468-2479.
- K.G. Papadokostaki Combined Experimental and Computer Simulation Study of the Kinetics of Solute Release from a Relaxing Swellable Polymer Matrix. II. Release of an Osmotically Active Solute J. Applied Polym. Sci. 92 (2004) 2458-2467.
- A. Stavropoulou, K.G. Papadokostaki, M. Sanopoulou, Experimental and Theoretical Study of the Release Kinetics of Propranolol Hydrochloride from PVA Matrices, J. Controlled Release 101 (2005) 313-315
- D.N. Soulas, K.G. Papadokostaki, A. Panou and M. Sanopoulou, Drug release from PDMS-based matrices: Observed and predicted stabilization of the release rate by three-layer devices, Ind. Engin. Chem. Res. 51 (2012) 7126-7136
- K.G. Papadokostaki, A. Stavropoulou, M. Sanopoulou, J.H. Petropoulos, An advanced model for composite planar three-layer matrix-controlled release devices. Part I. Devices of uniform material properties and non-uniform solute load, J. Membrane Sci. 312 (2008) 193-206.
- K.G. Papadokostaki, M. Sanopoulou, J.H. Petropoulos, An advanced model for composite planar three-layer matrix-controlled release devices. Part II. Devices of non-uniform material properties and a practical example, J. Membrane Sci., 343 (2009) 128-136.
- K.G. Papadokostaki, S.G. Amarantos and J.H. Petropoulos, Kinetics of Release of Particulate Solutes Incorporated in Cellulosic Polymer Matrices as a Function of Solute Solubility and Polymer Swellability. I. Sparingly Soluble Solutes, J. Applied Polym. Sci., 67 (1998) 277-287.
- K.G. Papadokostaki, S.G. Amarantos and J.H. Petropoulos, Kinetics of Release of Particulate Solutes Incorporated in Cellulosic Polymer Matrices as a Function of Solute Solubility and Polymer Swellability. II. Highly Soluble Solute,J. Applied Polym. Sci., 69 (1998) 1275-1290.
- K.G. Papadokostaki and M. E. Herouvim, Kinetics of Release of Particulate Solutes Incorporated in Cellulosic Polymer Matrices as a Function of Solute Solubility and Polymer Swellability. III. Moderately Soluble Solute,J. Appl. Polymer Sci., 84 (2002) 2028-2039.
- D.N. Soulas, M. Sanopoulou, K.G. Papadokostaki, A comparative study on the release kinetics of osmotically active solutes from hydrophobic elastomeric matrices, combined with the characterization of the depleted matrices, J. Appl. Polymer Sci., 113 (2009) 936-949.
- D.N. Soulas, K.G. Papadokostaki, Experimental investigation of the release mechanism of proxyphylline from silicone rubber matrices,J. Appl. Polymer Sci., 120 (2011) 821-830.
- A. Hasimi, A. Stavropoulou, K.G. Papadokostaki, M. Sanopoulou, Transport of water in polyvinyl alcohol films: effect of thermal treatment and chemical crosslinking Eur. Polymer J. 44 (2008) 4098-4107.
- D.N. Soulas, K.G. Papadokostaki, M. Sanopoulou, Silicone rubber films modified by ethylenoxy moieties: characterization and drug delivery properties, J. Applied Polym. Sci., 129 (2013) 874-883.
- D.N. Soulas, M. Sanopoulou, K.G. Papadokostaki, Hydrophilic modification of silicone elastomer films: thermal, mechanical and theophylline permeability properties, Mater. Sci. Eng. C: Appl. Biomat., 33 (2013) 2122-2230.
- A.I. Panou, K.G. Papadokostaki , P.A. Tarantili , M. Sanopoulou, Effect of hydrophilic inclusions on PDMS crosslinking reaction and its interrelation with mechanical and water sorption properties of cured films, Eur. Polym. J. 49 (2013) 1803-1810.
- A.I. Panou, K.G. Papadokostaki, M. Sanopoulou, Release mechanisms of semipolar solutes from poly(dimethylsiloxane) elastomers: Effect of a hydrophilic additive, J. Appl. Polym. Sci. 131 (2014) 9381-9388
- A.I. Panou, N. Mantes, K.G. Papadokostaki, M. Sanopoulou, Solute permeability of elastomeric films containing dispersed swellable hydrophilic particles: A composite material approach, Eur. Polymer J. 93 (2017) 471–479.
- M. Tsoka, P. Oikonomou, K. G. Papadokostaki, and M. Sanopoulou, Properties of Polydimethylsiloxane Modified by Blending with Polyvinylpyrrolidone and a Poly(ethylene oxide)-Poly(propyleneoxide) Triblock Copolymer, Ind. Eng. Chem. Res. 59 (2020) 5797−5807 .