Fundamentals of transport phenomena
Modelling of permeability properties of binary and three-phase polymer composites
The proper theoretical description of the permeability or analogous properties (thermal and electrical conductivity, electrical or magnetic permittivity, elastic modulus, etc.) of heterogeneous polymer systems is fundamental for proper material design in a variety of different applications such as immiscible polymer blends, block copolymers and semicrystalline polymers with impermeable crystalline regions.1-3 In recent years the development of nanocomposite materials have been used for barrier applications by incorporating impermeable platelets in polymeric phases and for gas separation membranes with embedded particles (zeolites , MOFs) highly selective for one or more gases. All these examples refer to composite materials consisting of at least two distinct well defined phases and the resulting permeability of the heterogeneous composite is related to the permeabilities of the pure constituting phases, the volume fractions of each component in the composite, as well as the system morphology (the shape and geometrical arrangement of the respective domains).
Maxwell' s classical equation for isometric particles embedded in a polymer medium, is rigorously valid only for very low fractional volumes of spherical particles, but is often used for data analysis up to much higher volume fractions.
We developed numerical and analytical tools using cubic lattices of congruent spheres or cubes to explore the applicability of Maxwell treatment (and the corresponding treatment of Wiener for anisometric particles) to high volume fractions of the embedded phase.2,4 Replacing the simple cubic lattice of spheres with an identical lattice of cubes, overcomes the limitations of the former due to the packing limit (since cubes can pack up to vA=1). This work proved that the practical application of the Maxwell, and the Wiener, models is much greater than is commonly believed.
The methodology was further applied to modeling three-phase composite medium systems, where the third phase is considered to be the polymer-particle interphase, exhibiting permeability properties differing substantially from those of the bulk polymer matrix. This effect is of practical importance and should be taken into account in modeling, especially in the case of nanocomposites, due the high surface to volume ratio of the nanoparticles. The practical importance of our modeling approach was tested against experimental data on gas permeability data.5
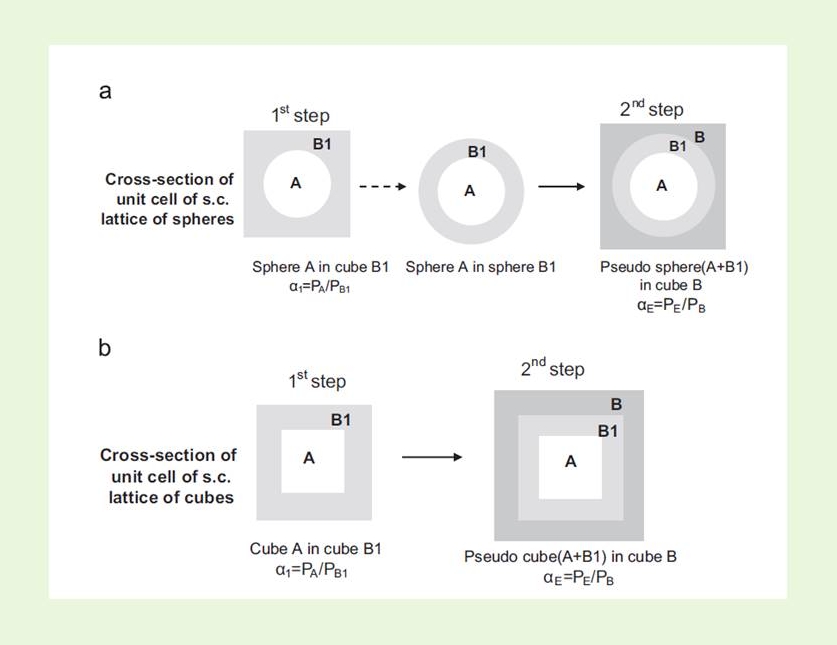
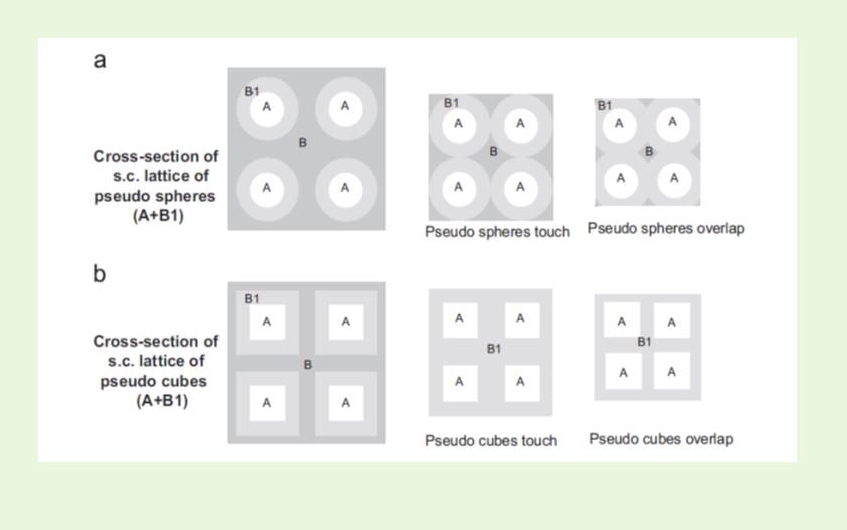
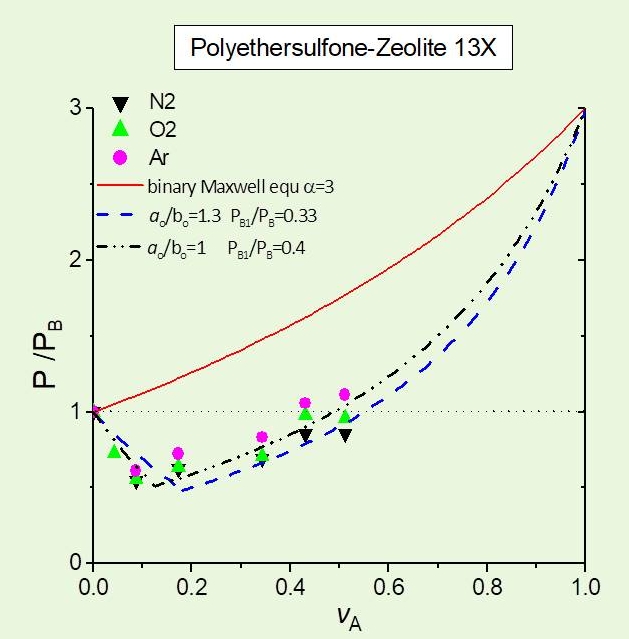
- Non-Fickian sorption kinetics in glassy polymers.
Sorption kinetics of swelling agents (organic vapour or liquids) in glassy polymer systems exhibits a variety of deviations from normal Fickian behaviour (t1/2 law) with the extreme kinetic behavior of Case II kinetics (t law).
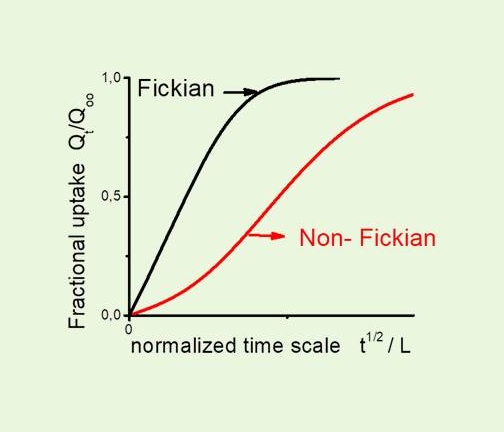
Our work in this field includes systematic and extensive experimental studies of sorption and diffusion of micromolecular species in polymeric films in order to establish a variety of non-Fickian kinetic features and to get insight on the underlying physical causes by the aid of appropriate models. The macroscopic models we applied and also tested against experiment were initially developed by J.H. Petropoulos6-8, and are based on the effect of intrinsic bulk polymer properties, leading to delayed (viscous) swelling and to build-up and decay of differential swelling stresses.9-11
A brief account of this work is given below.
The non-Fickian behaviour is attributed to either(i) slow viscous relaxations of the swelling polymer. In general polymer–organic vapor systems usually exhibit concentration-dependent diffusion behavior, with a diffusion coefficient D, being a strong function of the concentration of sorbed penetrant due to penetrant-induced swelling and plasticization of the polymeric medium. For rubbery polymeric systems, characterized by high segmental mobility (glass transition temperature Tg of the system well below the experimental temperature T), the relevant swelling process is fast relative to diffusion and the resulting sorption kinetics is Fickian. For glassy polymeric systems (characterized by Tg>T), on the other hand, completion of the local swelling process may be sufficiently slow (delayed swelling) due to the low segmental mobility of the glassy state. Delayed swelling rate (rate parameter β) may be comparable with, or slower than, that of diffusion (rate parameter Dt/L2); thus yielding time dependent non-Fickian sorption kinetics (which typically produce “S-shaped” or “two-stage” absorption √t plots).
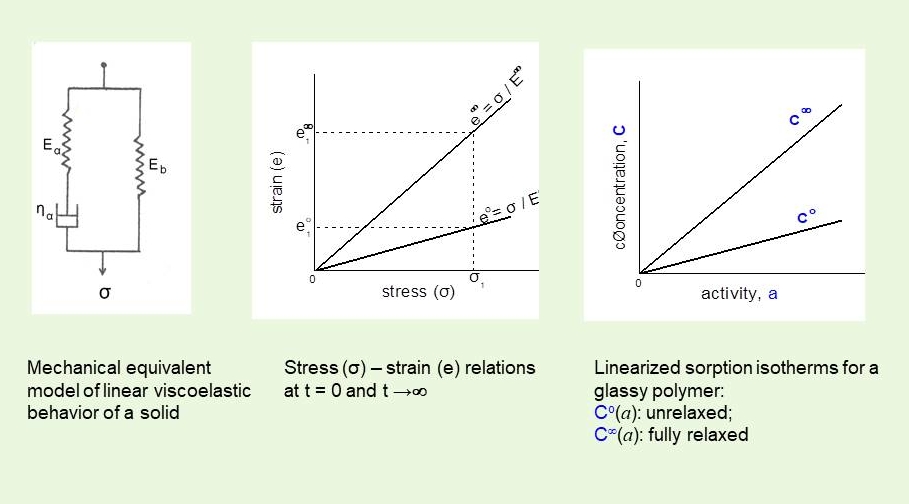
There is a close analogy between the instantaneous (elastic) and delayed (viscous) strain (deformation) response of a viscoelastic solid under mechanical stress and the instantaneous and delayed volume swelling strain (concentration) of a glassy polymer in response to an osmotic stress (activity). The initial sorption isotherm (Co vs a) reflects fast very local, , microstructural relaxations needed to accommodate incoming penetrant molecules and the final isotherm (C∞versus a) corresponds to slow longer range macromolecular relaxations(taken from ref [6])
or (ii)differential swelling stresses generated by the constraints imposed on local swelling during sorption. During diffusion along the thickness direction X, due to non-uniform concentration (C) along X, compressive DSS (-f) are exerted by the glassy core on the outer swollen regions and extensive DSS (-f) on the dry core. During sorption, the stresses in the swollen region may decay due to the more uniform concentration profile, or due to the viscoelastic response of the swelling polymer (i.e. plasticization effects on Young modulus and stress relaxation effects on the swollen parts). These stresses affect the penetrant uptake kinetics as well as the longitudinal swelling of the polymer film.
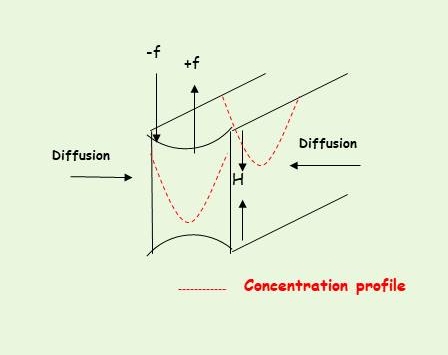
The major operating mechanism in a non-Fickian specific system depends on the experimental conditions, and work of our group has focused on identifying which mechanism prevails and under which experimental conditions.
The methodology adopted consists of
(i) investigating the predicted kinetic behavior on the basis of theoretical models developed for both mechanisms , in parallel with
(ii) detailed experimental study of penetrant sorption from the vapor or the liquid phase.
Sorption from the vapor phase12-16
Vapor absorption experiments yield a broad spectrum of kinetics, which has been studied extensively. The experimental polymer –organic vapor systems were studied in vacuum sorption apparatuses under conditions of (i) high or low concentrations of sorbed penetrant (ii) high or low concentration range (iii) high or low thickness of polymer films. Strict experimental protocols were applied due to the history -dependence behavior of glassy polymer systems (aging phenomena). In order to analyse theoretically our data, sorption kinetics were studied in parallel to the kinetics longitudinal swelling of the films, and supplemented by the mechanical behavior of the polymer- penetrant systems12.
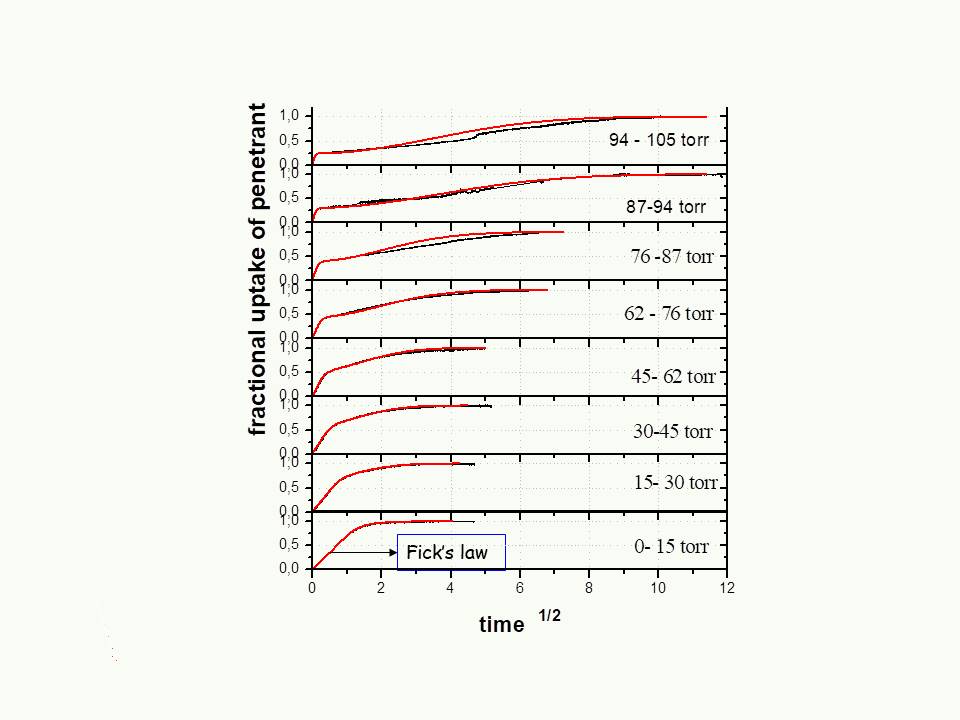
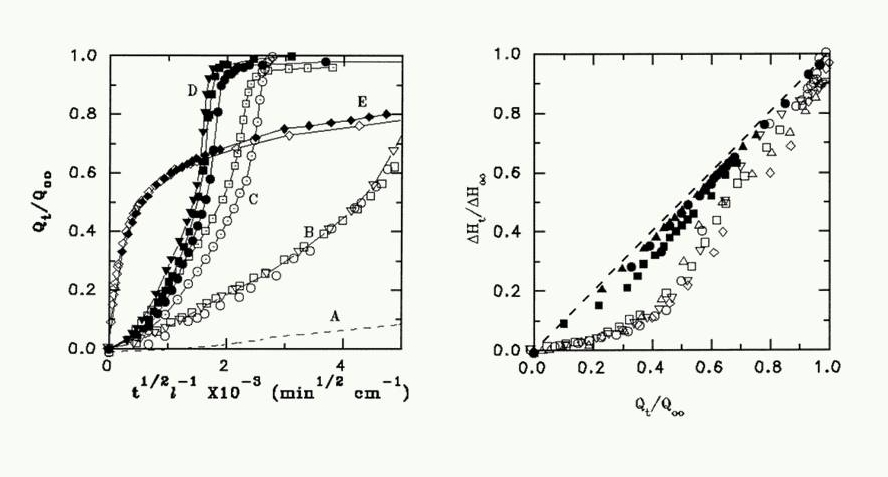
Main achievements of this work were
- experimental validation of theoretical predictions on a qualitative or semiquantitative level
- in conjunction with models’ predictions, we developed diagnostic criteria which allow us to determine whether the viscous relaxation mechanism or the differential swelling stresses one, or both, is responsible for the non-Fickian kinetic behavior in any polymer-penetrant system. This work provided a complete and final answer to the problem of identification of the two mechanisms described above.
- The diagnostic method was first applied to the cellulose acetate-acetone system,12 which exhibited various non-Fickian features depending on the experimental conditions. The main outcome of this analysis is that the said behavior cannot be fully understood without invoking both mechanisms.
- The above conclusion was found to be applicable to other systems studied, i.e. cellulose acetate –methanol13, polymethylmethacrylate-ethyl acetate14 and polymethylmethacrylate-methanol15. This work lead to a reform of the generally accepted interpretation of non-Fickian kinetics of micromolecular penetrants in glassy polymers, which was previously based exclusively in terms of viscous swelling relaxations.
Sorption from the liquid phase17-20
Under these conditions, sorption process covers one single concentration range, determined by the chemical affinity between polymer and liquid penetrant. For a particular polymer this range can be modified by changing the liquid penetrant.
Detailed studies on the mechanisms of non-Fickian kinetics in model systems were performed by sandwiching the polymer film between clamped glass plates to allow penetration only across the exposed edges of the films and applying different optical techniques (see schematic below). The information derived on penetration rates, concentration profiles and mechanical stresses imposed on the swelling polymer film, provided detailed data for experimental testing of the theoretical models on non-Fickian behavior. Experiments in cellulose acetate 17-19 included (i) a series of penetrants of varying polarity to study the kinetics of penetration in a range of concentrations up to complete dissolution of the polymer (ii) chemical modification of polymer’s polarity by partial hydrolysis and (iii) modification of polymer’s mechanical properties by uniaxial orientation of macromolecules along the film. The same techniques have been applied, in conjunction with the theoretical analysis of Case II kinetics, for the experimental investigation of Case II kinetics of methanol penetration in polymethyl methacrylate film.20
An example of this work is given below. The transition of penetration kinetics from Fickian to Case II is observed when CH2Cl2 penetrates a Cellulose Acetate film normal or parallel to the macromolecular orientation, is simulated on a semi quantitative level by setting the parameter characterizing the deformability of the polymer under the swelling stresses , high for penetration normal to the orientation axis and low for penetration parallel to the axis, under otherwise identical conditions.
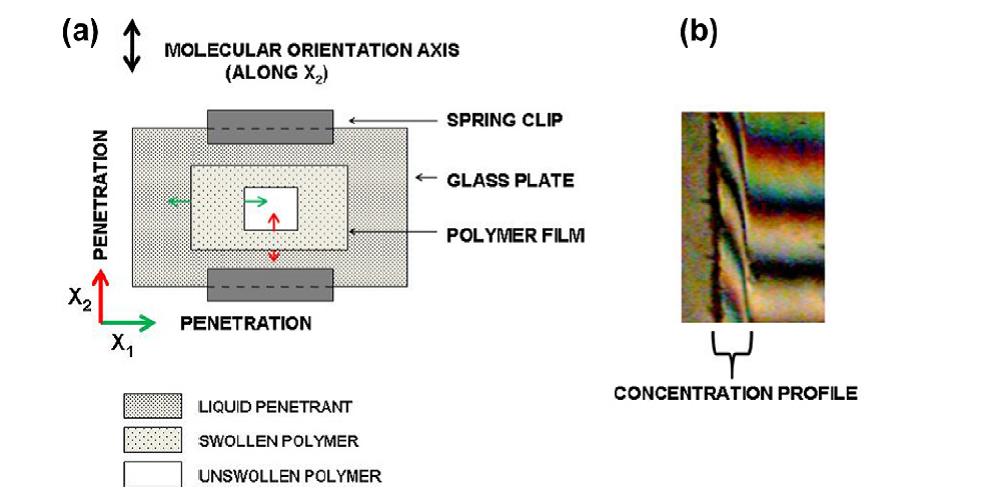
A polymer film is sandwiched between clamped glass plates to allow penetration only across the exposed edges of the films, immersed in the liquid penetrant. By optical microscopy at right angles to the plates the kinetics of inward-moving penetration fronts and corresponding outward-moving swelling fronts are recorded. Scanning optical densitometry and microinterferometry are applied to provide concentration profiles in the swollen polymer and birefringence measurements provide information on the mechanical stresses acting on the area.
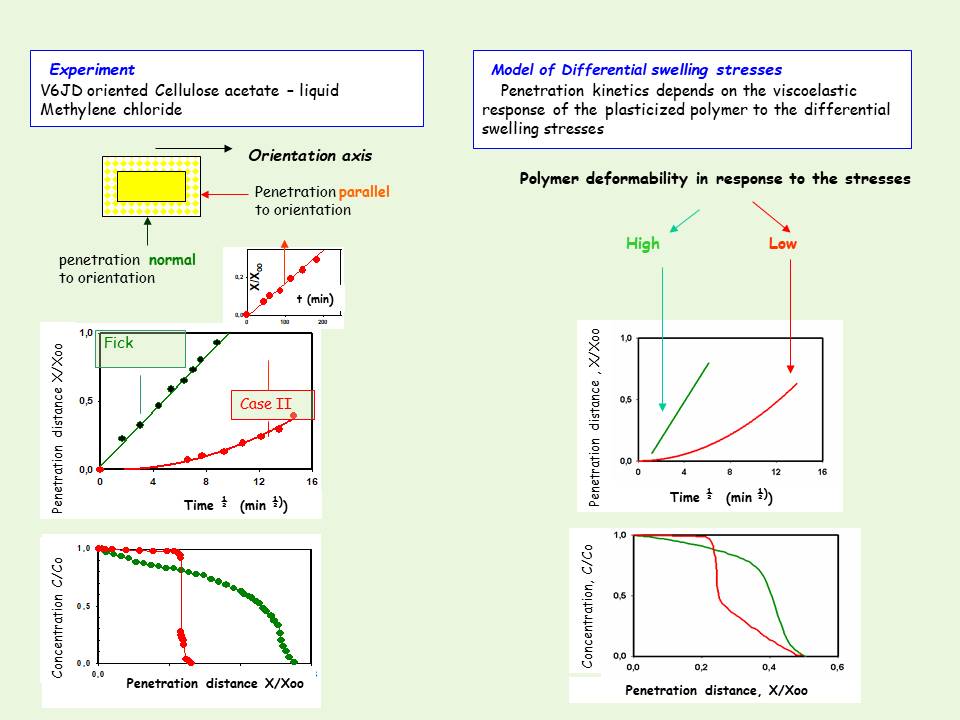
Penetration normal to the axis of preferred molecular orientation was characterized by relatively fast penetration (as well as fast swelling rate) obeying Fickian kinetics (t1/2 law), and typical Fickian concentration-dependent profiles , but penetration parallel to orientation axis was slower, characterized by Case II (t law) kinetics and concentration profiles. The basic characteristics of this kinetic behavior have been successfully simulated by setting, the parameter characterizing the deformability of the polymer under the swelling stresses , high for penetration normal to the orientation axis and low for penetration parallel to the axis, keeping all other parameters the same (taken from ref [9]).
Finally the transport properties of silmethylene homo-polymers and random copolymers21,
silicone rubber22 and in polyvinyl alcohol23 were also studied.
Selected references
- J. H. Petropoulos, A Comparative study of approaches applied to the permeability of binary composite polymeric materials. J. Polym. Sci.,Polym. Phys. Ed. 1985, 23, 1309−1324
- M. Minelli, F. Doghieri, K.G. Papadokostaki, J.H. Petropoulos, A fundamental study of the extent of meaningful application of Maxwell's and Wiener's equations to the permeability of binary composite materials. Part I: A numerical computation approach, Chem. Eng. Sci. 104 (2013) 630-637.
- J.H. Petropoulos, K.G. Papadokostaki, M. Minelli, F. Doghieri, “On the role of diffusivity ratio and partition coefficient in diffusional molecular transport in binary composite materials with special reference to the Maxwell equation”, J. Membrane Sci.,456 (2014) 162-166..
- K.G. Papadokostaki, M. Minelli, F. Doghieri, J.H. Petropoulos, A fundamental study of the extent of meaningful application of Maxwell's and Wiener's equations to the permeability of binary composite materials. Part II: A useful explicit analytical approach, Chem. Eng. Sci. 131 (2015) 353-359.
- J.H. Petropoulos, K.G. Papadokostaki, F. Doghieri, M. Minelli, A fundamental study of the extent of meaningful application of Maxwell's and Wiener's equations to the permeability of binary composite materials. Part III: Extension of the binary cubes model to 3-phase media, Chem. Eng. Sci. 131 (2015) 360-366.
- J. H. Petropoulos, Interpretation of anomalous sorption kinetics in polymer-penetrant systems in terms of a time-dependent solubility coefficient. J Polym Sci Polym Phys 1984;22:1885–900.
- J. H. Petropoulos, Effect of a stress-dependent solubility coefficient on sorption kinetics in penetrant-polymer membrane systems. J. Membrane Sci 1984;18:37–51.
- J. H. Petropoulos, Application of the transverse differential swelling stress model to the interpretation of Case-II diffusion kinetics. J Polym Sci. Polym Phys Ed 1984;22:183–9.
- J.H. Petropoulos, M. Sanopoulou, , K.G. Papadokostaki, “Physically insightful modeling of non-Fickian kinetic regimes encountered in fundamental studies of isothermal sorption of swelling agents in polymeric media” Eur. Polym. J. 47 (2011),2053-2062
- J. H. Petropoulos, M. Sanopoulou, K. G. Papadokostaki “Beyond Fick: How best to deal with non-Fickian behavior in a Fickian spirit” Diffusion Fundamentals III, August 23-28, 2009, Athens, Greece
- M. Sanopoulou and J.H. Petropoulos, “Systematic Analysis and Model Interpretation of Micromolecular Non-Fickian Sorption Kinetics in Polymer Films”, Macromolecules, 34 (2001) 1400-1410.
- M. Sanopoulou, Roussis PP, Petropoulos JH. A detailed study of the viscoelastic nature of vapor sorption and transport in a cellulosic polymer. I. Origin and physical implications of deviations from Fickian sorption kinetics. J Polym Sci Part B: Polym Phys 1995;33:993–1005.; II . Sorption and longitudinal swelling kinetic correlations. J. Polym Sci Part B: Polym Phys 1995;33:2125–36.
- M. Sanopoulou and J.H. Petropoulos, “Sorption and Longitudinal Swelling Kinetic Behavior in the System Cellulose Acetate- Methanol”, Polymer, 38 (1997) 5761-5768.
- J.P. Boom and M. Sanopoulou, “Interval Sorption Kinetics in the System Poly(methyl methacrylate)-Methyl Acetate”, Polymer, 41 (2000) 8641-8648
- V. Dimos, M. Sanopoulou, “Determination of sorption kinetic parameters using the relaxation dependent solubility model in the methanol vapor – poly(methyl methacrylate) system” , J. Appl. Polym. Sci., 100 (2006) 2278-2288
- D. F. Stamatialis, D. Ν., M. Sanopoulou “Mechanisms of non-Fickian micromolecular diffusion in glassy polymer films: Analysis of experimental sorption and concurrent dilation kinetics in the light of a Differential Swelling Stress model”, Diffusion Fundamentals III, August 23-28, 2009, Athens, Greece
- M. Sanopoulou and J.H. Petropoulos, “Phenomenology and Mechanisms of Unidimensional Stress-Dependent Micromolecular Transport in a Stiff-Chain Polymer. I Unoriented Cellulose Acetate”, J. Polym. Sci., Part B: Polym. Phys., 30 (1992) 971-982
- M. Sanopoulou and J.H. Petropoulos, “Phenomenology and Mechanisms of Unidimensional Stress-Dependent Micromolecular Transport in a Stiff-Chain Polymer. II. Uniaxially Oriented Cellulose Acetate”, J. Polym. Sci., Part B: Polym. Phys., 30 (1992) 983-992
- D.F. Stamatialis, M. Sanopoulou and J.H. Petropoulos, “Phenomenology and Mechanisms of Unidimensional Stress-Dependent Micromolecular Transport in a Stiff-Chain Polymer. ΙΙΙ. Effect of Modification of the Polymer”, J. Polym. Sci., Part B, Polym. Phys., 35 (1997) 2593-2607
- M. Sanopoulou, Stamatialis DF, Petropoulos JH. Investigation of Case II diffusion behavior. I. Theoretical studies based on the relaxation-dependent solubility model. Macromolecules 2002;35:1012–1020 ΙI. Study of the Poly(methyl methacrylate)-Methyl Alcohol System by Two-Beam Microinterferometry, Macromolecules, 35 (2002) 1021-1027.
- A. Alentiev, I. G. Economou, E. Finkelshtein, J. Petrou, V. E. Raptis, M. Sanopoulou, S. Soloviev, N. Ushakov, Y.Yampolskii “Transport Properties of Silmethylene Homo-polymers and Random Copolymers: Experimental Measurements and Molecular Simulation”, Polymer, 45 (2004) 6933-6944
- D.F. Stamatialis, Wessling M, Sanopoulou M, Strathmann H, Petropoulos JH. Analysis of the kinetics of vapor absorption/ desorption in/from silicone rubber and cellulose acetate membranes in the presence of stagnant boundary layers. J Membrane Sci 1997;125:165–75
- A. Hasimi, Stavropoulou A, Papadokostaki KG, Sanopoulou M.Transport of water in polyvinyl alcohol films: effect of thermal treatment and chemical crosslinking. Eur Polym J 2008;44:4098–107