Mössbauer Spectroscopy of Magnetic Compounds
Mössbauer Spectroscopy (MS) is a powerful tool for the study of materials at the atomic level.
The technique uses the ultrafine sensitivity of nuclear transitions to their surroundings in the form of valence, magnetic state and external magnetic fields, as well as crystal symmetry.
The group has many years of experience in the application of Mössbauer spectroscopy to Rare-Earth (RE) Transition-Metal (TM) compounds, oxides, ores, magnetic nanoparticles, and thin films.
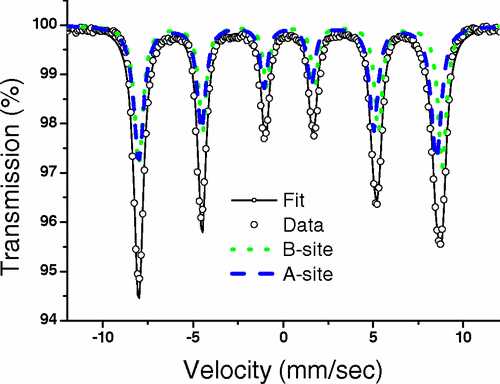
Recent published work on core-shell maghemite-silica nanoparticles demonstrated the power of MS to reveal the magnetic state with the np’s and the effects of magnetostatic magnetic interactions resulting from proximal nanoparticles. Theoretical modelling using Monte Carlo simulations were validated by magnetization and MS data. The existence of additional reversal mechanisms that lower the energy barrier for magnetic reversals is supported by Mössbauer data taken over a renage of temperature, from 4.2K to 300K, indicating a faster decrease of the hyperfine magnetic field value at the surface relative to the core, for all silica shell thicknesses, as predicted by the Collective Magnetic Excitations (CME) model of Mørup and Topsøe.
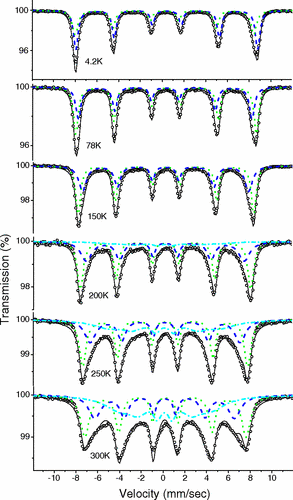
Research studies on the novel Fe16N2 phase have also used MS. Fe16N2 is a compound with giant saturation magnetization approaching or exceeding that of rare-earth-based permanent magnets. The abundance of its constituent elements and low-cost synthesis make it a candidate to replace expensive rare-earth-based permanent magnets The phase was synthesized from Fe flakes by surfactant-assisted high energy ball milling. The Fe flakes are reduced under forming gas (Ar/H2), followed by nitridation at low temperatures under ammonia (NH3) gas.
Mössbauer spectroscopy is especially useful in detecting iron phases in nanoparticles which may be underestimated by XRD analysis. It also gives information on the degree of crystallization of iron phases. In these studies we observed all the samples to contain metallic iron (α-Fe), Fe16N2 , and a small amount (≤6% of total iron) of a non-ferromagnetic iron phase (NM) revealed as a broad central peak which may originate from fine superparamagnetic particles that lack the critical size to become ferromagnetic. The parameters of the Fe16N2 phase are in good agreement with those published for thin film samples indicating a well-crystallised phase. The Fe16N2 phase content is approximately constant at 40-45% of the total iron. Fe3N is present in some samples, the fitted parameters being agin in good agreement with published work. The amount of metallic iron present decreases with annealing temperature with metallic iron being progressively replaced by iron in the form of Fe3N with increasing annealing temperature. The Mössbauer data is in good agreement with the XRD analysis for the phase compositions, and also demonstrates the absence of any significant iron oxidation. This work clearly shows the usefulness of the MS technique in obtaining accurate information on materials with dimensions at the nanoscale.
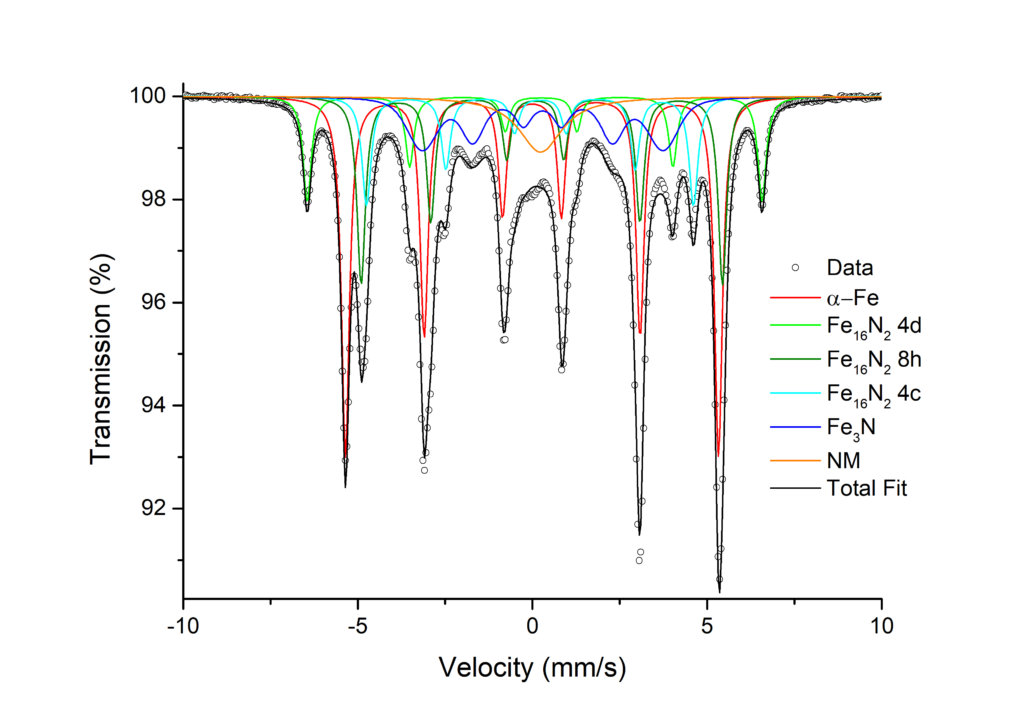
Samples produced by Dr V. Tzitzios, responsible for the Research Activity on Chemical Synthesis of Multi-Functional Nanomaterials, have been studied also. These are iron carbide nanoplatelets with the orthorhombic Fe3C structure synthesized following a simple liquid chemical approach. The formation of the carbide phases was shown to depend on the presence of a long chain diol and the reaction temperature. The simple methodology for producing iron carbide nano-platelets has promising application in the biomedical and catalyst industries. The catalytic properties of iron carbides are also of interest in the Fischer-Tropsch process which is used for producing liquid fuels from a variety of sources. The limiting factor in utilizing iron carbide nanomaterials for these applications is the lack of simple methods for producing high-quality single-phase material, and the ability to tailor their size and shape for a given application. Confirmation of the iron carbide phases and structural characterization was made by X-ray diffraction (XRD) and Mӧssbauer spectroscopy while particle morphology was characterized by transmission electron microscopy (TEM) and HR-TEM and the magnetic properties were measured with magnetometry (VSM). In the study we identified the Fe3C phase and its phase percentage by Mӧssbauer spectroscopy, achieving a colloidal synthesis of iron carbide nano-platelets with an Fe3C content of 79% and a magnetization of 139 emu/g at 30 kOe applied field. To the best of our knowledge, this was the first single-step liquid chemical synthesis of Fe3C nanoplatelets produced without the use of Fe(CO)5 iron precursor.
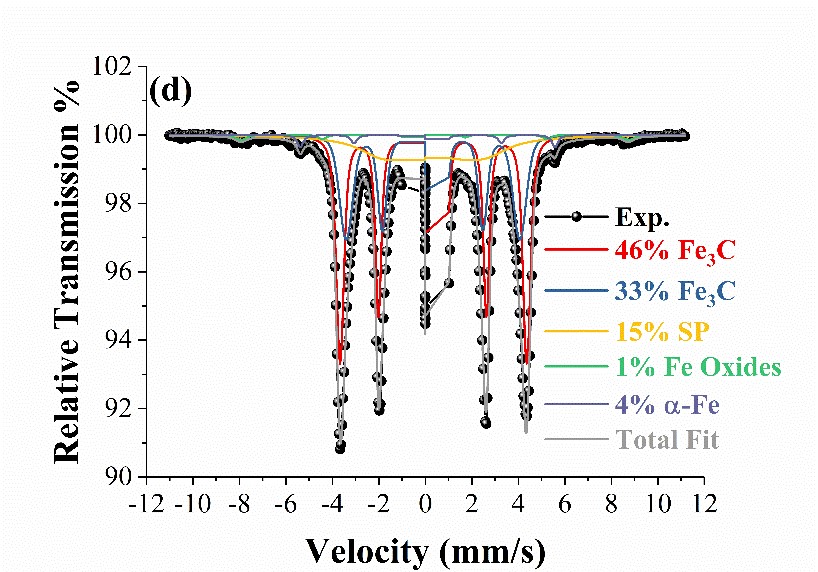
Mӧssbauer spectroscopy is also used to perform difficult structural assessments of the L10 phase in FePt alloys being able to distinguishing the tetragonal L10 and cubic fcc FePt phases. These phases are also studied with the Research Activity on Magnetic Thin Films and Metamaterials. The tetragonal L10 phase is readily identified in Mӧssbauer spectra by its large quadrupole interaction, eQ. L10 highly ordered FePt nanostructures were successfully synthesized using a direct one-step liquid phase chemical approach. The enhanced ordering was achieved with the presence of bismuth additives in the reaction mixture, the ferromagnetic nanoalloys revealing high coercivity without any post annealing step. The effect of bismuth addition was studied extensively and the synthesized nanostructures were characterized by a plethora of techniques including TEM, STEM elemental mapping, XRD, and Mӧssbauer spectroscopy for the structural and morphological characterization
For the sample prepared at 320 oC this tetragonal phase subspectrum makes up approximately 60% of the total spectral area of the room temperature spectrum, while the cubic site accounts for 30% of the spectral area. For the sample prepared at 360 oC the tetragonal has increased to 74% of the total spectral area, with the cubic site now accounting for 16% of the spectral area. A third subspectrum, a nonmagnetic doublet, with just 10% of the spectral area, is also required for an acceptable fit of the experimental data in both samples at room temperature. In the spectrum of the 360 oC sample obtained at 80K we see that the nonmagnetic component has decreased to just 5% of the spectral area with a corresponding increase in the area of the cubic phase, while the tetragonal phase area remains constant. This is in keeping with low magnetic anisotropy (fcc phase) nanoparticles, superparamagnetic at room temperature, becoming magnetic at low temperature. The Mӧssbauer Spectroscopy thus confirms the formation of the L10 phase at low reaction temperatures using a bismuth additive.