Microfluidics Design & Simulation
Computational studies are carried out guiding the design of highly performant microfluidic devices and predicting/understanding the device performance. We have focused mainly on microfluidics accommodating micromixing, DNA amplification, and rare cell separation, i.e., devices that constitute crucial components of lab-on-a-chip systems with widespread applications in disease diagnosis and food safety.
We have proposed novel designs of micromixers, which have been realized, evaluated experimentally and applied to biochemical reactions, such as DNA digestion. Extensive computational studies are being also performed to design both static chamber and continuous flow microPCR devices, investigating the effect of the substrate material stack and thickness on the power consumption, the time required for the completion of amplification and DNA concentration. Fast and low energy consuming microPCR devices were designed and means were investigated for the improvement of the device performance. For microPCR devices integrated with microheaters and preferably industrially manufactured on printed circuit boards (PCBs), it became evident that a decrease in the thickness of the PCB stack, an increase in the power applied to the microheaters, the use of active cooling, and the integration of a copper layer between the microheaters and the microPCR chamber decreases the PCR protocol duration and the energy consumption and optimizes the temperature uniformity of the device.
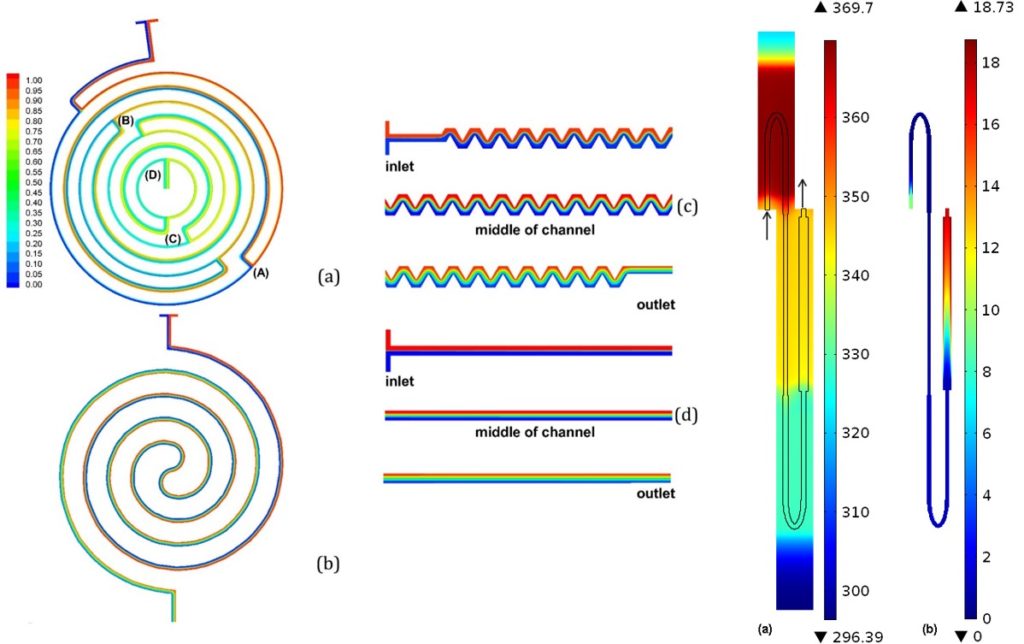
ritical for the efficiency of the DNA amplification is the residence time of DNA molecules in each one of the three temperatures of the PCR. We performed detailed numerical calculations of the DNA residence time distributions, within an acceptable temperature range for denaturation, annealing, and extension, for the first time in the literature, which provided useful information regarding the actual on-chip PCR protocol and justified the maximum volumetric flow rate for successful DNA amplification. The calculations indicated that the shortest amplification time is achieved when the device is operated at its enzyme kinetic limit (i.e., extension rate).
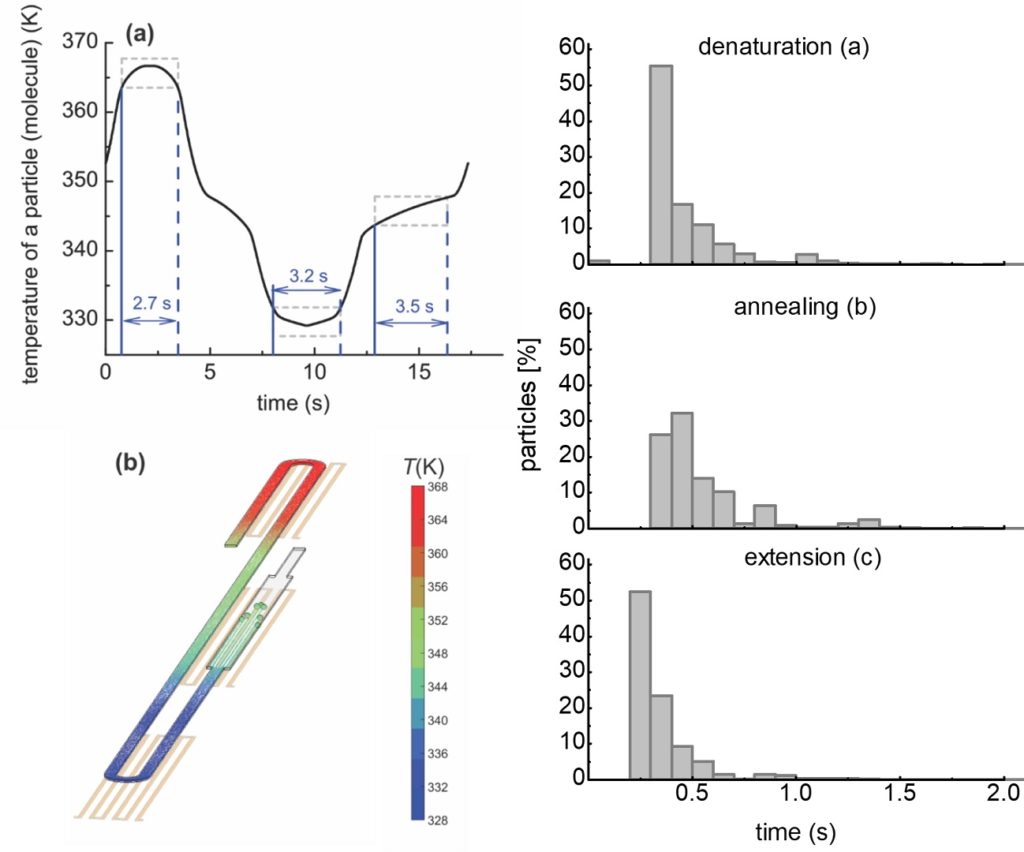
The effect of the heat loss mechanisms and parameters on the heating and cooling rates of microPCR devices as well as on their power and energy requirements was investigated. In detail, a new effective model for the convective heat losses was proposed, where the heat transfer coefficient (h) is derived as a function of the surface temperature. The new model not only compares satisfactorily with a detailed 3d framework, but also entails a 10-fold lower computational cost.
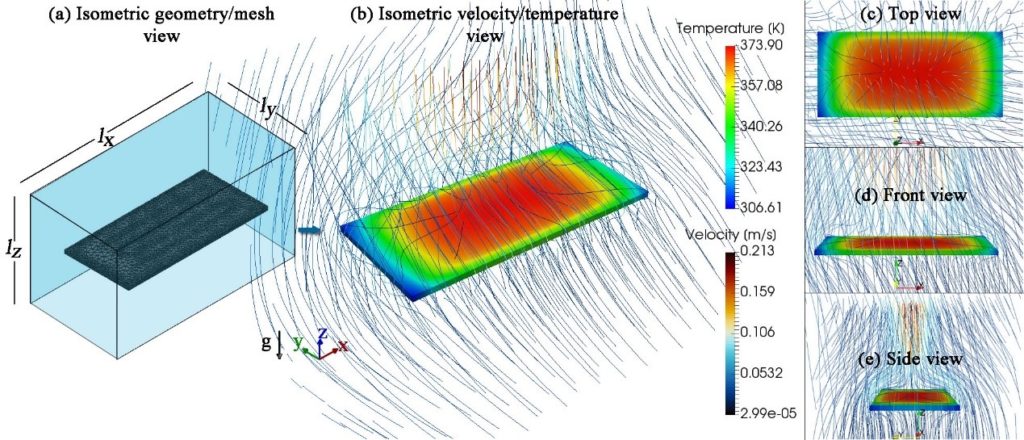
Calculations are currently in progress aiming at the design of a novel closed-loop microPCR device, where very fast, flexible and efficient DNA amplification protocols can be performed. In addition, computational studies in microfluidics for rare cell (inertial or immunochemical based) separation are progressing and maturing enough to be soon realized and evaluated.
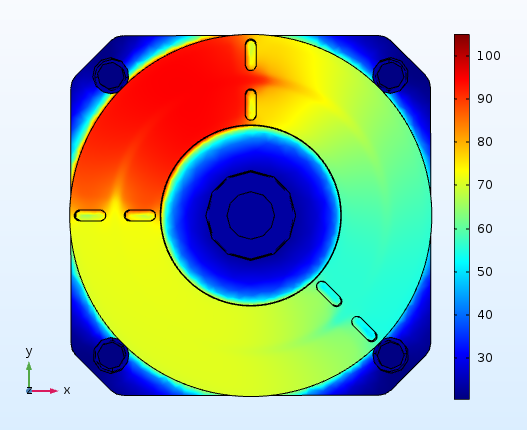