Spirotetronate polyketides
Humans have utilized natural products long before they understood their structure or mechanism of action. A representative example of this is the use of willow bark to treat fever, inflammation and general pain. In modern times, natural products have been the primary source for the discovery of new antibiotics. Thus, over 70% of the clinically used antibiotics are natural products or have been derived from natural products. Although this has been a successful strategy, unfortunately, the long-term effects of wide spread antibiotic use over the last few decades have led to the Resistance Era. To fight back antibiotic resistance it is crucial to discover novel antibiotics. Our laboratory aims to synthesize natural products with, among other, antibiotic activity. In particular, we are interested in synthesizing the family of the abyssomicins, a marine class I spirotetronates (Figure 1).
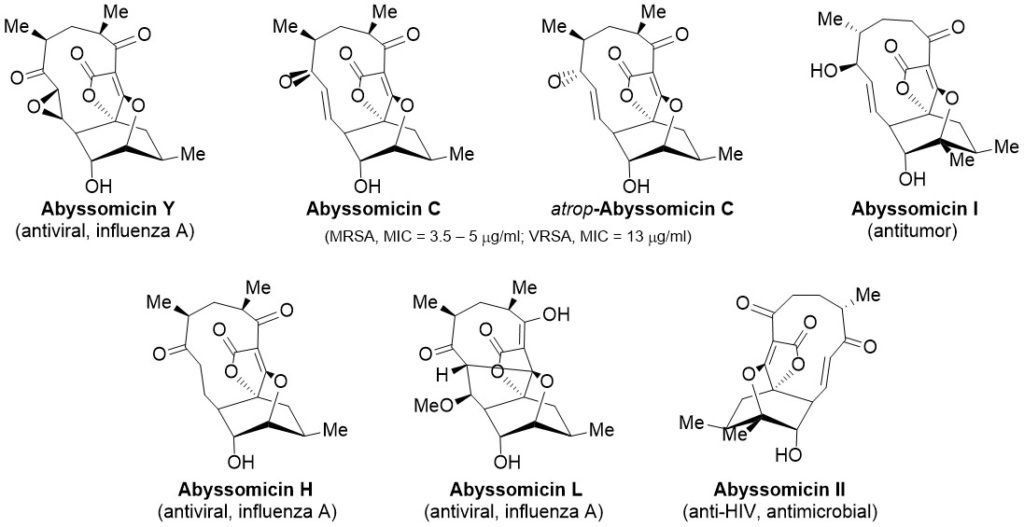
Figure 1. The family of abyssomicins.
The interest in the abyssomicins pharmacophore was fueled by its antibacterial activity targeting mycobacteria and Gram-positive bacteria like S. aureus, including MRSA and VRSA.1 Notably, in addition to their antibacterial activities, this family also includes natural products which exhibit latent HIV reactivation and antitumor activities.
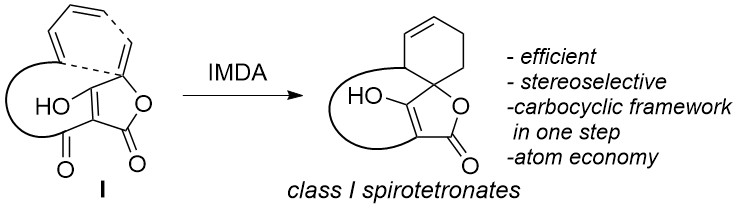
Figure 2. Biosynthesis of class I spirotetronate polyketides.
Biosynthetically, they derive from suitably substituted tetronic acid derivatives (I) via a stereoselective intramolecular Diels–Alder (IMDA) reaction, which establishes all stereogenic centres and the carbon framework of these molecules in one step. Consequently, this impressive and atom-efficient transformation has been utilized in many powerful bioinspired synthetic approaches.2
Intrigued by the effectiveness of their biosynthesis and the fact that previous biomimetic approaches, albeit impressive, suffered from serious issues, we aimed at the modification of these methodologies to increase the overall yield towards the key intermediate 3, which is known to lead effectively to abyssomicin C (1), its atrop-isomer (2) and its IMDA-precursor (4; Figure 3).3
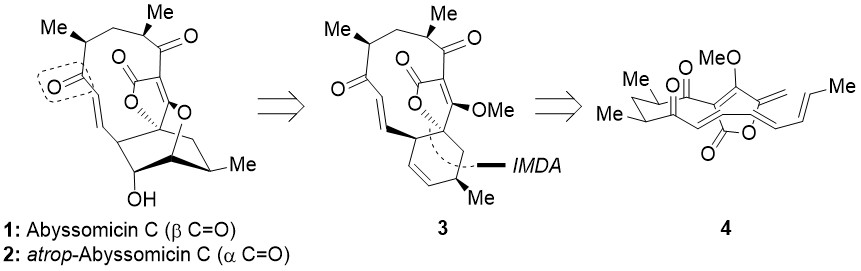
Figure 3. Key intermediates towards abyssomicin C (1) and its atrop-isomer (2).
Our manoeuvres augmented the yield to 3, which was thus prepared in an uncomplicated and efficient manner.[4]
Based on the results of this research, current efforts towards the synthesis of other members of this family and analogues and studies for further enhancement of the biomimetic approach are underway. This research has been the subject of two MSc theses, implemented and completed in our laboratory, and an ongoing PhD thesis under the supervision of Dr V. Vidali.
References
- J. Riedlinger, A. Reicke, H. Zähner, B. Krismer, A. T. Bull, L. A. Maldonado, A. C. Ward, M. Goodfellow, B. Bister, D. Bischoff, R. D. Süssmuth, H.-P. Fiedler, J. Antibiot. 2004, 57, 271–279.
- (a) M. H. Lacoske, E. A. Theodorakis, J. Nat. Prod. 2015, 78, 562−575; (b) A. Braddock, E. A. Theodorakis, Mar. Drugs 2019, 17, 232−247.
- (a) C. W. Zapf, B. A. Harrison, C. Drahl, E. J. Sorensen, Angew. Chem. Int. Ed. 2005, 44, 6533–6537; (b) B. Snider, Y. Zou, Org. Lett. 2005, 7, 4939–4941; (c) E. A. Couladouros, E. A. Bouzas, A. D. Magos, Tetrahedron 2006, 62, 5272–5279.
- V. P.Vidali, A. Canko, A. D. Peroulias, E. T. Georgas, E. Bouzas, J. M. Herniman, E. A. Couladouros, Eur. J. Org. Chem. 2020, 4547–4557.